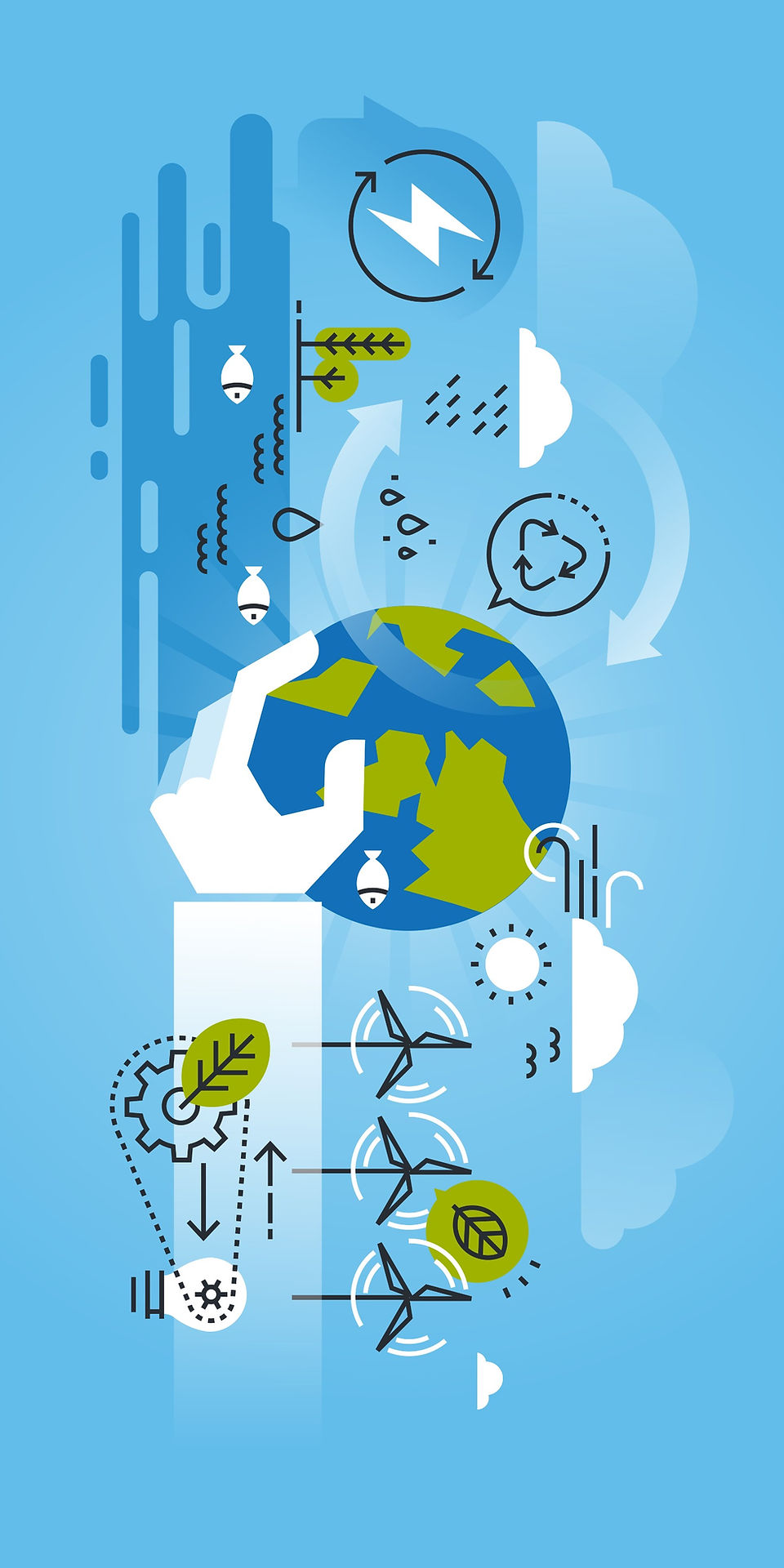
Sustainable solar energy conversion and storage system using earth-abundant materials
Research Achievement: During my graduate study, I have investigated the synthesis of nanostructured graphitic carbon nitride (g-CN), a highly ordered graphitic polymer with surface amine functionalities and semiconductor property. Specifically, I looked into the nanocasting approach and molecular cooperative assembly via hydrogen bonding for the introduction of nanostructure.[1] Such nanostructured carbon nitrides found its application in various fields: 1) abundant surface amine and nitrogen functionalities for adsorption of heavy metal ions, organic acids, and enzymes, 2) homolytic bond cleavage above 650°C evolving reductive CNx species for the synthesis of mesoporous ordered metal carbides and nitrides, and 3) intrinsic semiconductor-like property for the label-free optical detection of trace amount of heavy metal ions (<100nM).[2]
Extended knowledge of g-CN was obtained from the research at UCSB. I focused on the optimization of electronic/electrical conductivity, minimization of charge trapping and the dynamics of substrate catalysts support interactions for enhanced photo- and electro-catalytic activities of g-CN in hydrogen evolution from water and Li-S/Air batteries.[3, 4] A significant change in the work was to design the molecular cooperative assembly of precursor triazine materials that 1) increase the light absorption ability, 2) can be fine-tuned to make more favourable electronic band structures, and 3) provides efficient redox electron communication of the delocalized aromatic molecules for the desired redox reactions of the resulting g-CN materials.[4, 5]
Grand Challenge: Based on knowledge that I have obtained so far from graduate and postdoctoral studies, I aim to develop a platform for sustainable synthesis of energy-rich organic molecules from CO2 and/or water, using integrated, artificial photosynthetic membranes consisted of 1) light harvesting earth-abundant organic semiconductor and 2) molecular or nanoparticle electro-catalysts that do not utilize noble metals and that are highly active for the synthesis of fuels and useful chemicals.
Background: Fossil fuels account for more than 80% of world primary energy consumption in 2013.[6] Although reserves of fossil fuels are being diminished and their combustion causes the climate change by evolving green house gases including CO2, global consumption of fossil fuels and thus CO2 emission are expected to inevitably grow further due to recent high levels of anxiety about nuclear power and an ever-growing population. This triggers the search for renewable, clean energy sources such as sun light.[7] Also, the use of CO2 as sustainable chemical feedstocks, not based on oil, are highly demanded for the sustainability of chemical enterprise.[8] Both factors point to an increased need for efficient, sustainable solar-to-chemical energy conversion/storage systems using CO2.
The reduction of CO2 to useful chemicals initiated by solar energy could be an attractive way to store renewable energy sources into chemical bonds and reduce harmful gas emissions into the environment. To this end, there have been extensive research efforts for the photocatalytic CO2 conversion using inorganic/organic semiconductor materials.[9] These studies, however, reveal that the artificial photosynthesis is still impractical due to the low yield, which can be attributed to 1) low utilization of visible light, 2) instability of semiconductor, 3) inefficient charge separation and backward reaction on semiconductor, 4) insufficient thermodynamic driving force for redox reactions and 5) disharmony between redox reactions in which multi-electrons and -protons take part.
Research Goals: The general objective of the proposed research is to develop an efficient, integrated system for sustainable reactions generating chemical fuels and electricity, for example, solar driven water splitting and CO2 conversion as well as electrocatalytic oxygen reduction (ORR) and evolution (OER). This will be achieved by the crystal engineering of triazine precursors for the fine-tuning of optoelectronic properties and the control of the multi-electron transfer processes at the interfaces. The synthetic breakthroughs will be coupled with the physical characterization to develop a fundamental understanding of the system and with the demonstration of reactivity as photo- or electro-catalyst. I will focus on the synthesis of metal-free polymer semiconductor, i.e. g-CN, with wide visible light absorption, high specific surface area, retarded charge recombination and sufficient electronic band energies to drive sustainable photocatalytic reactions. The development of synthetic method and catalytic performance evaluation, involving either isolated or composite nanoscale materials, will be implemented as outlined in the specific aims below.
References
[1] Y. S. Jun, W. H. Hong, M. Antonietti, A. Thomas, Advanced Materials 2009, 21, 4270-+; K. Kailasam, Y. S. Jun, P. Katekomol, J. D. Epping, W. H. Hong, A. Thomas, Chemistry of Materials 2010, 22, 428-434.
[2] E. Z. Lee, Y. S. Jun, W. H. Hong, A. Thomas, M. M. Jin, Angewandte Chemie-International Edition 2010, 49, 9706-9710.
[3] J. Park, Y.-S. Jun, W.-r. Lee, J. A. Gerbec, K. A. See, G. D. Stucky, Chemistry of Materials 2013, 25, 3779-3781; K. A. See, J. A. Gerbec, J. Young-Si, F. Wudl, G. D. Stucky, R. Seshadri, Advanced Energy Materials 2013, 3, 1056-1061.
[4] Y.-S. Jun, J. Park, S. U. Lee, A. Thomas, W. H. Hong, G. D. Stucky, Angewandte Chemie International Edition 2013, 52, 11083-11087.
[5] Y.-S. Jun, E. Z. Lee, X. Wang, W. H. Hong, G. D. Stucky, A. Thomas, Advanced Functional Materials 2013, 23, 3661-3667.
[6] Bwk 2002, 54, 24-25.
[7] J. Manassen, D. Cahen, G. Hodes, A. Sofer, Nature 1976, 263, 97-100.
[8] T. Inoue, A. Fujishima, S. Konishi, K. Honda, Nature 1979, 277, 637-638.
[9] S. C. Roy, O. K. Varghese, M. Paulose, C. A. Grimes, Acs Nano 2010, 4, 1259-1278; S. Navalon, A. Dhakshinamoorthy, M. Alvaro, H. Garcia, Chemsuschem 2013, 6, 562-577; M. Cokoja, C. Bruckmeier, B. Rieger, W. A. Herrmann, F. E. Kuhn, Angewandte Chemie-International Edition 2011, 50, 8510-8537.